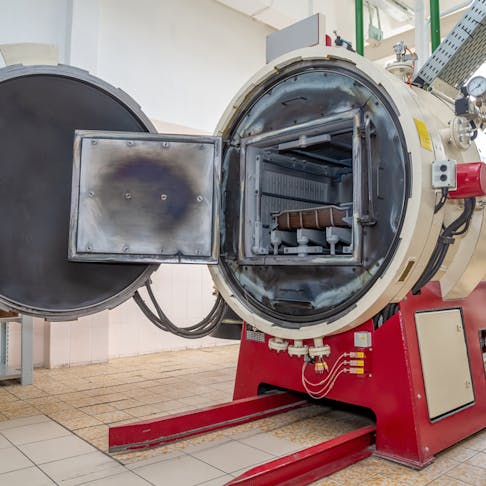
Annealing vs. Tempering: The Key Differences Between Them
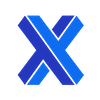
Annealing and tempering are closely related processes that use the application of heat and a slow cooling process to adjust the microstructure of various metals, to partially or fully relieve the effects of hardening in the crystalline matrixes of the metals. Related and similarly named processes also have applications in other classes of materials—particularly in glass and the more crystalline polymer materials.
What Is Annealing?
Annealing is a heat treatment process used to alter the properties of metals and other materials, typically to make them softer, more ductile, and less brittle. It involves heating the material to a specific temperature and then cooling it slowly in a controlled manner, to manipulate the crystalline structure.
The process can be performed in precise or approximate ways. Precision annealing involves slow cooling in a furnace in which the temperature ramps down over a programmed cycle. Process annealing is a less rigorous form that simply allows air cooling. The annealing process choice, functional temperature, and cooling duration depend on the material type, its previous processing history, and the desired properties.
How Does Annealing Work?
Annealing subjects the material to a carefully controlled heating and cooling sequence. The goal of annealing is to achieve specific changes in the material's microstructure and properties.
The material is heated to a specific annealing temperature, above the material's critical temperature, at which its crystal structure becomes less stable. The rate of heating is controlled to avoid thermal shock, in materials that are susceptible to this. After reaching the annealing temperature, the material is held at that temperature for a specific duration to ensure that heating is complete and uniform and that slow crystalline adjustments have sufficient time to complete. The material is then cooled slowly and uniformly. The cooling rate is a critical aspect of annealing. Slow cooling is often achieved by turning off the furnace and allowing the material to cool within the furnace or in a controlled atmosphere. The slow cooling allows for the formation of desired microstructures and the reduction of internal stresses.
What Is the Purpose of Annealing?
Annealing serves various purposes in materials processing and manufacturing. It is a near-universal heat treatment technique in steels and some other metals. Annealing softens materials that have become hard or brittle due to previous manufacturing processes, such as: heat/quenching, cold working, or machining. The treatment increases the ductility and malleability of materials, making them easier to work with in subsequent processing steps. Annealing relieves internal stress that has accumulated in the material during prior operations. It promotes the formation of new, smaller, and more regular/consistent grains in the material's microstructure, which enhances various mechanical properties of the material. Annealing helps achieve a more uniform composition throughout the material, particularly in alloys, by allowing the diffusion of elements within the material. Annealed materials are generally easier to machine and form, reducing tool wear and improving throughput. In materials like ferrous alloys, annealing can improve electrical conductivity or magnetic permeability.
What Is the Importance of Annealing?
Annealing plays a crucial role in materials processing and manufacturing, and its importance is underscored by critically important benefits such as: workability, relieving of internal stresses, and improved machinability.
It is the most widely applied heat treatment process that serves to optimize material properties. Annealing also improves manufacturing efficiency and ensures the reliability and quality of finished products across various industries, including: aerospace, automotive, electronics, and construction.
What Are the Benefits of Annealing?
The benefits of annealing are varied and their importance in an individual context can often be related to the nature of the parts being annealed and the post-heat-treatment processes they must undergo. These advantages are:
- Improved workability.
- Relief of internal stresses.
- Enhanced ultimate tensile strength (UTS) and toughness.
- Anisotropic uniformity.
- Improved machinability.
- Better response to subsequent heat treatments.
- Reduced brittleness.
- Improved electrical conductivity and magnetic permittivity.
- Consistent inter-batch properties and improved quality control.
What Are the Drawbacks of Annealing?
While annealing offers several advantages, it also has some potential drawbacks and limitations as listed below:
- Can be time-consuming, especially when heavier gauge materials require slow and controlled cooling.
- The high temperatures required for annealing can result in significant energy consumption/cost, especially in large-scale industrial settings.
- Annealing furnaces and equipment have size limitations, making it challenging to anneal very large components. Raw materials in large lengths can be continuously processed in linear conveyor furnaces, however.
- During heating, materials generally develop surface scale or oxidation, requiring additional finishing processes to remove this.
- Generally has a limited impact on electrical conductivity or magnetic permeability.
- The cost of equipment, energy, and labor associated with annealing can be a drawback.
What Is Tempering?
Tempering is a heat treatment process used primarily on steel and its various alloys. It alters their mechanical properties, primarily to increase toughness and reduce brittleness. The tempering process involves heating a previously hardened material to a specific temperature and then cooling it in a controlled manner.
The consequence of tempering is material with improved toughness, reduced brittleness, and a balance between hardness and ductility which can be adjusted by controlling the tempering temperature and dwell times. This process is widely used in industries in which strong and tough steel components are required, such as in: the manufacturing of springs, tools, machine components, and blades/cutters.
How Does Tempering Work?
Tempering involves a transformation of the material’s crystalline microstructure to deliver specific adjustments to the mechanical properties. The process adjusts the proportions and distribution of crystalline regions and types of crystal matrixes within the material, to exploit the relative benefits of different microstructures.
Hardened steels generally possess a martensitic structure. Martensite is a highly stressed and brittle crystalline structure formed during rapid quenching. It has a supersaturated arrangement of atoms and is characterized by a body-centered tetragonal lattice. First, the material is heated to a temperature below its hardening temperature but still relatively high. The fine-tuning of properties results from the finesse with which a range of target temperatures can be reached and held for the dwell time. This heating initiates changes in the martensitic structure. As the material is heated, some of the martensite transforms into austenite, a face-centered cubic (FCC) matrix. Austenite is a more stable phase at higher temperatures and has a more regular arrangement of atoms compared to martensite, reducing its tendency to fracture.
During the dwell period (rest at elevated temperature), atomic diffusion occurs. Carbon atoms, in particular, diffuse within the lattice, leading to the precipitation of small carbides within the crystal lattice. The cooling phase of tempering allows the material to retain some of the austenite, but it also reverts some of it back to a transformed martensitic structure. The resulting microstructure is called tempered martensite, which has a more refined and less stressed lattice compared with the original martensite.
The tempered martensite microstructure offers improved toughness and ductility compared to the preexisting, brittle martensite. The fine carbide precipitates within the structure act as barriers to dislocation and crack propagation, enhancing the material's mechanical properties.
What Is the Purpose of Tempering?
The primary purpose of tempering is to optimize the mechanical properties of steel and its alloys and to adjust the balance between hardness and toughness. It increases the toughness of materials by recovering a controlled degree of the ductility that is lost in heat/quenching hardening. Tempering reduces brittleness and improves the ability to withstand impact and dynamic loads without fracturing. It also reduces the hardness of heat/quenched steels.
While hardness is valued, full hardness carries a burden of fracture risk that suggests compromise properties are more valuable in many cases. Tempering refines the microstructure of the material, promoting the formation of altered crystalline forms and small carbide precipitates within the lattice. This microstructure adjustment enhances both strength and ductility. Tempering can relieve internal stresses that accumulate during heat/quench or work hardening, reducing the risk of distortion, warping, or fracture in the finished product. It makes materials easier to machine, weld, and form, reducing tool wear and easing manufacturability. Tempering ensures the consistency of material characteristics, vital for quality control and inter-batch repeatability.
What Is the Importance of Tempering?
The importance of tempering lies in its ability to optimize the mechanical properties of materials, primarily steel and its alloys, to achieve a balance between hardness and toughness.
Tempering is essential in materials used in engineering and manufacturing because it allows materials to be fine-tuned to meet the specific demands of their intended use. It ensures that materials are appropriately strong, tough, and resilient for the intended application, across industries from automotive and construction to aerospace and tool manufacturing.
What Are the Benefits of Tempering?
Tempering brings well-understood benefits to the materials it is applicable to:
- Enhanced toughness (reduced brittleness).
- Microstructure refinement.
- Stress relief.
- Customization of Properties.
- Improved workability (ductility and malleability).
- Selective control of hardness.
- Property consistency/repeatability.
- Optimized use of materials.
What Are the Drawbacks of Tempering?
While tempering is a valuable heat treatment process with many advantages, it also has some potential drawbacks and limitations:
- Achieving the desired combination of properties through tempering can be a skilled and slow process, requiring careful control of temperature and time.
- The size of the material or component being tempered is restricted by the capacity of available tempering furnaces.
- The heating process during tempering produces surface discoloration or scaling which generally requires additional finishing processes.
- The heating and tempering process requires considerable energy.
- The need for restored toughness necessitates a reduction in hardness, as the two properties are interlinked in the atomic structure.
What Is the Role of Annealing and Tempering in Steel Microstructure?
Annealing and tempering are two crucial heat treatment processes that play distinct roles in modifying the microstructure of steel, which in turn affects its mechanical properties.
Annealing promotes the formation of a more stable, refined, and less stressed microstructure. During the heating phase, austenite forms a more regular arrangement of atoms. While the steel is held at the annealing temperature, atomic diffusion and migration occur. Carbon atoms diffuse within the lattice, precipitating carbide particles within the matrix. The cooled material retains some of the austenite but also reverts some of it back to a martensitic structure that is more regular and less stressed than that of the pre-annealed state. The resulting tempered martensite has a more balanced combination of hardness and toughness than the heat/quenched or work-hardened martensite.
Tempering, on the other hand, begins with the material in its hardened state, typically martensite, a body-centered tetragonal lattice with elevated hardness and high brittleness. Tempering aims to reduce “excess” hardness and increase toughness. During tempering, the martensitic structure is transformed to a primarily austenitic structure and includes the condensation of fine carbide precipitates within the matrix. The cooling phase of tempering allows the material to retain a proportion of austenite, but it also reverts some of it back to tempered martensite. The proportions can be fine-tuned by tempering temperature and dwell time. The result is a tunable degree of improved toughness and balanced hardness and ductility.
How Do Annealing and Tempering Differ in Process?
Annealing is a low-control process, in which the target state is unitary, irrespective of the starting condition of the material. That is, if the temperature of a part is raised to a sufficient level, i.e., the annealing temperature, and then the part is cooled slowly, the fully annealed state will result.
There is no variation in the target for this, i.e., a partially annealed state is rarely required. The fully annealed state is one in which:
- All regions of the part have the maximum possible crystal size.
- All internal stresses are relieved.
- All traces of any prior heat treatment or hardening have been eradicated from the crystalline structure.
Tempering, on the other hand, is a precise and quantified process in which a part is first hardened, generally by heat/quench processing, and then the temperature is raised to an annealing level that is moderately controlled, within ranges of 100–200 °C. The heat is maintained for a critical and predetermined dwell time to allow the part to become completely uniform in temperature and for the microstructural alterations to commence and proceed fully throughout. The part is then allowed to cool in open air at room temperature, to complete the revision of the microstructure.
The degree of tempering depends on the desired properties sought from the process and the initial state of hardening of the part, making this a much more complex process with a spectrum of outcomes that require precision control. In some regards similar to an annealed structure, the tempered material will show:
- A slight to significant reduction in hardness.
- A slight to extreme increase in toughness and ductility.
- Some degree of retention of the hardened microstructure, from moderate to virtually unmodified.
- Reduced, but not fully eased, residual stress.
What Are the Temperatures Used in Annealing and Tempering?
The stable temperature the part is raised to is the only control mechanism used in annealing. Once the annealing temperature is achieved or exceeded, the part can be allowed to cool to room temperature, in an open-air environment.
In tempering, the applied heat is the primary control mechanism whereby the degree of temper is defined. Selecting a lower target tempering range will deliver partial tempering, which is sufficient to mildly alter the residual stresses within the part, slightly reduce the hardness, and slightly improve the fracture performance of the part. Some internal stresses and brittleness will remain, and the part will be close to the hardness achieved in heat/quenching. Selecting higher tempering levels will increase the ductility and fracture resilience of the part and reduce the hardness further. Precise selection of the temper point temperature allows precise tuning of material properties and a high degree of repeatability in the qualities of the resulting parts.
In a more primitive form of tempering control, the achieved temperature of the part can be estimated from the color of the oxide film that results—signifying increasing levels of temper from light straw to gold to brown to purple to violet to dark blue to light blue to gray.
However, the surface temperature is not representative of the inner temperature, on thicker sections. It is for this reason that a temperature-controlled furnace is likely to give more consistent results.
How Do the Applications of Annealing and Tempering Differ?
Annealing serves the one purpose of removing all heat-treatment or work-hardening-imposed microstructural changes from a part. The material is returned to its fully ductile/malleable state. This allows for shaping and forming to proceed with minimum risk of microstructural or macro damage.
Annealing is widely applied as a process for various metals such as: nickel and its alloys, stainless steels, carbon steels, copper and its alloys, and titanium and its alloys. It is an aid to ease manufacturing and finds application in various metals that both can and cannot undergo hardening by heat/quenching. Work-hardened metals suffer embrittlement and hardening by work being done to them.
Tempering is a fine adjustment process that can tune the properties of previously hardened materials, allowing a wide spectrum of outcomes from near fully hard and brittle (typical of a file) to fairly ductile and with somewhat reduced hardness (typical of a spring or a screwdriver tip). Tempering is, therefore, often seen as a finishing process for many parts made in steel, to fine-tune their properties once shaping is complete.
Tempering can also be a fine-tuning process that serves to remove residual stresses prior to machining, so parts as-machined will not distort when cut, allowing heat treatment to be performed prior to cutting. This is typical of injection mold tool bolsters/cavities which are generally shaped after hardening/tempering, using EDM or wire cut methods and high-speed carbide cutters.
Which Is More Expensive, Annealing or Tempering?
Of the two processes, tempering is considerably more costly both in time and skills.
Annealing is a simple process that uses a brief elevation of temperature to a level above the critical and glass transition temperature of the material, followed by slow cooling. Tempering requires a precise and very uniform heating of the part; a dwell time at the elevated temperature that depends on part size and section thickness; and a slow cooling in open air. This is more technically demanding and takes longer than annealing.
When To Use Annealing Instead of Tempering?
Annealing is a process used to remove any unwanted, thermally applied heat/quench effects, work hardening, or a combination of the two forms of microstructure change. The treatment is used to return the material to its relaxed and most workable state, to allow forming processes to be completed with less effort and risk of fracture.
Tempering is applied to adjust material properties after a hardening process, generally to remove some of the detrimental effects that hardening has wrought upon the part or material.
How Do Annealing and Tempering Differ in Materials?
Annealing has wide application across most metals. In general, it is a method used to either prepare raw material for forming or as an intermediate treatment that allows the removal of work hardening during processing, to allow further forming stages to be undertaken with less risk of fracture. It is applicable to many metals, from aluminum to tantalum, from most stainless steels to copper.
Tempering is a much more narrowly applicable method that is applied most commonly to steel alloys of various varieties to relieve some of the negative effects of heat/quench hardening. It is also used in beryllium-copper, and to a lesser extent in brass, bronze, aluminum, copper, and even some cast iron materials.
Summary
This article presented annealing vs. tempering, explained each of them, and discussed their key differences. To learn more about annealing and tempering, contact a Xometry representative.
Xometry provides a wide range of manufacturing capabilities and other value-added services for all of your prototyping and production needs. Visit our website to learn more or to request a free, no-obligation quote.
Disclaimer
The content appearing on this webpage is for informational purposes only. Xometry makes no representation or warranty of any kind, be it expressed or implied, as to the accuracy, completeness, or validity of the information. Any performance parameters, geometric tolerances, specific design features, quality and types of materials, or processes should not be inferred to represent what will be delivered by third-party suppliers or manufacturers through Xometry’s network. Buyers seeking quotes for parts are responsible for defining the specific requirements for those parts. Please refer to our terms and conditions for more information.
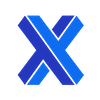